Gamma rarely accompanies alpha decays
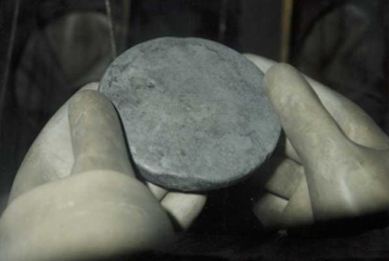
Plutonium isotopes are alpha emitters
Twice as dense as lead, plutonium has a silver-like aspect in a metallic state. The plutonium isotopes are alpha emitters except for plutonium-241. Alpha emitters are not dangerous unless they are inhaled or ingested. The layer of our skin is able to stop them. In the case of plutonium, penetrating gamma rays that often accompany radioactive decays are missing or have remarkably low energy. This explains why this technician can handle a piece of plutonium with simple gloves.
© DR
It is surprising that one can hold with hands a sample of plutonium like the one in the photograph. How can one hold such a radioactive element in hands, even with thick gloves? Are we not exposed to gamma rays?
The military-grade plutonium sample in the photograph is predominantly made of plutonium-239, an element nearly 200,000 times more radioactive than uranium. The alpha rays that it emits are very toxic, but they are stopped by the smallest thickness of material. Gloves protect perfectly from these rays by avoiding any contact.
There remain the gamma rays which accompany the alpha. In the case of plutonium-239, the gamma emitted is not very energetic and above all it is rare: 1 gamma emitted for 3000 decays! The handling should not be prolonged, but the relatively low intensity of the radiation allows time to take a photograph.
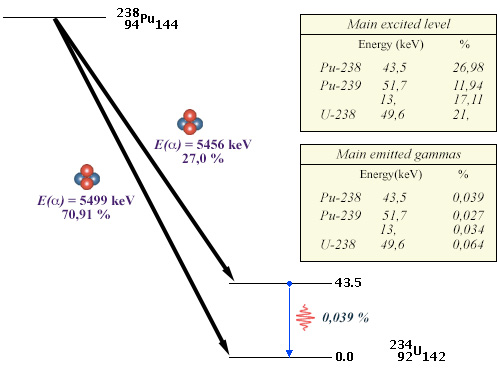
Low excitations levels and rare gamma decays
Example of the plutonium-238 decay diagram. If 27% of the decays lead to an excited level, the 43,5 keV excitation energy of this level is low compared to the 5456 keV of the alpha ray. Desexcitation occurs mainly by internal conversion. The emission of a gamma of 45.6 keV occurs only 3.9 times in 10,000. Similar characters are found in alpha decays, such as those of plutonium-239 and uranium-238.
© IN2P3 (Source BNL data base)
Rare gammas with low energy characterize the decays of plutonium-238 (used as an energy soure in spatial probes), plutonium-239 and uranium-238, as shown in the example above. These two properties are specific to most heavy alpha emitting nuclei (an exception is that of uranium-235).
This is the case with uranium and its descendants, which explains why the exposure of living beings to rays emanating from rocks is kind for our greater good. This is also the case for plutonium isotopes. This is the reason for the use of plutonium-238 as an on-board energy source for distant space probes.
For the three quoted nuclei cited, the gamma energy is at most 50 keV and the frequencies less than 1 per 1000. The energies and frequencies are much lower than what can be observed with beta decays. For instance, the energy of the most frequent gamma ray emitted by iodine-131 is 364 keV, its frequency 81.5%. For cesium-137, the energy of the main gamma is 662 keV, its frequency 85%!
For the minor actinides present in nuclear waste, the gamma energies remain low, but the frequencies are higher. This is the case, for example, with americium-241 that emits a 59 keV gamma with a frequency of 36%. Despite this americium gamma, the minor actinides play a minor role in the intense gamma radiation coming from a vitrified waste package.
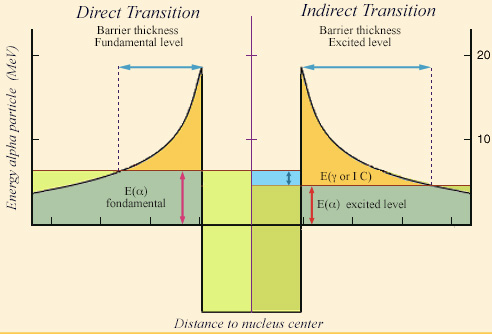
Tunnel effect with an excited level
During a transition from an excited energy level of the nucleus, the kinetic energy of the alpha particle is decreased by the excitation energy (right). To escape the attraction of the nucleus, the alpha particle will have to cross a greater thickness of the “potential barrier” which retains it. The probability of observing the alpha particle outside, which decreases very quickly with this thickness, becomes negligible when the energy of the excited level is important.
© IN2P3
The low energy and scarcity of such gamma rays can be explained by the mechanism of alpha decays, the tunnel effect. To get out of the nucleus, the alpha particle must escape the attraction of other nucleons. It must cross a barrier (the “potential barrier“). The very long radioactive half-lives of most alpha emitters demonstrate that this barrier is already very difficult to cross. If in addition, the alpha particle is to be accompanied by a gamma, its kinetic energy is diminished by the energy of that gamma. The thickness of the barrier to be crossed will be increased and the alpha particle will have even more difficulty to cross it in order to leave the nucleus. This explains the rarity of accompanying gammas.
The emission of such a gamma means that the alpha decay has left the nucleus in an excited state. In order for the emission of gamma not to be too rare, this excitation energy must be low. Therefore, only energy levels of a few tens of keV are observed in alpha decays (NB: Internal conversion often prevails over the emission of a gamma as a means of de-excitation when the energy to be evacuated is low).
Other articles on the subject « Radioactive nuclei »
Map of Nuclei
Map of stable and unstable nuclei The progress made in our understanding of the subatomic world o[...]
Stability Valley
Beta decay : nuclei getting slimmer to achieve stability The nucleus mass is related to its inter[...]
Nuclear Forces
Three nuclear forces and their hierarchy Three types of force act alongside each other inside a n[...]
Mecanisms of Radioactivity
The way radioactivity works and its origins The impressive range of half-lives that exist, extend[...]
α decay : tunnel effect
Particle and wave: an effect of quantum mechanics The great age of uranium and thorium nuclei tha[...]
β decay : weak forces
The forces which allow a nucleus to emit beta electrons Beta decay (β) and electronic capture cha[...]
Weak Forces
A special and fascinating fundamental interaction A third force is at work in the nucleus next to[...]
The Nucleosynthesis
Primordial and stellar nucleosynthesis The Universe hasn’t always existed, It was born a li[...]
Nucleosynthesis (continued)
Mechanisms of atomic nuclei formation Most of the nuclei of atoms that make up our daily life wer[...]