Neutrons resulting from Nuclear fissions
Radioactive decays with neutron emission are extremely rare. In our environment, the main natural source is the bombardment of the atmosphere by cosmic radiation. The neutrons thus produced interact with the nitrogen nuclei of the air and produce carbon-14, a natural radioactive nucleus.
To emit spontaneously neutrons, nuclei should have a large excess of neutrons or be very heavy. This is the case of californium-252, a super heavy nucleus having 14 more nucleons than uranium. Californium-252 is used as a source of neutrons, especially for starting reactors. The spontaneous fission of heavy nuclei is also a source of natural neutrons, but with very low probabilities, of the order of one millionth for natural elements such as thorium-232, uranium 238 and 235.
Why, in these conditions, should we be interested in neutrons and their becoming in matter? Although the role of natural neutrons is minimal, they are the agents of nuclear fission, of its military and civil applications, from atomic bombs to energy-generating reactors.
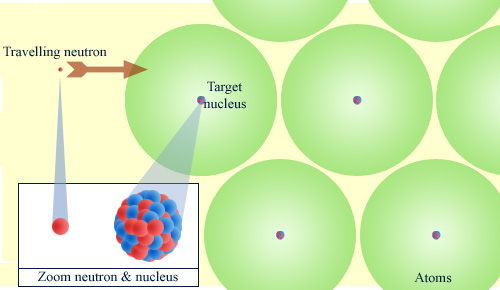
Mainly vacuum for neutrons
When it travels through matter, a neutron sees only the nuclei of atoms. The electronic clouds are transparent to it. To make the figure understandable, the neutron and the nuclei were enlarged, but at atom scale they look punctual. The neutron almost only encounters vacuum, which explains why the neutron is particularly penetrating.
© IN2P3
The neutrons of nuclear fissions …
The behaviour of neutrons in matter and more particularly in the core of reactors is the object of the science of Neutronics.
A free neutron is an unstable particle, with a 10.2 minutes half-life. For this reason it is quasi absent in our environment. In a dense medium, a neutron is captured long before it decays, sometimes triggering a nuclear reaction when the nucleus becomes unstable.
Neutrons are neutral particles only sensitive to nuclear forces. Therefore they travel readily and untouched through the electron cloud of atoms encountered. However they may interact strongly with their nuclei. But the nuclei are very small and the neutron radiation is more penetrating than gamma radiation. The most common interactions are simple collisions. However during these collisions the neutron can be captured and then trigger a nuclear reaction.
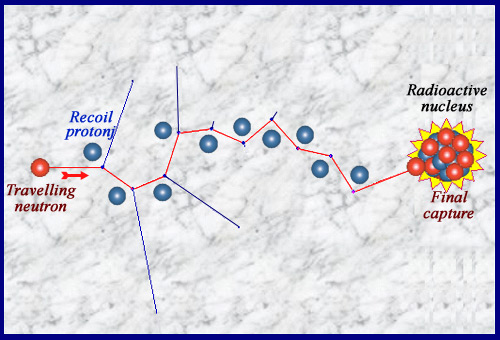
A billiards game …
Travelling throguh matter, neutron undergoes “elastic” collisions on nuclei. It transmits its energy to the nuclei recoiling (the path of the neutron, which does not interact between collisions, is invisible). This slowdown is the fastest in a hydrogenated matter where recoil nuclei are protons with a mass equal to the neutron. These electrically charged recoil nuclei are the one that ionize and produce side effects. The neutron is generally finally captured by a nucleus that can become radioactive: the material is activated. In special cases such as uranium-235 or plutonium-239, the capture triggers the explosion of the nucleus, a fission.
© IN2P3
A series of collisions then finally a capture …
The neutrons resulting from a fission have an energy of the order of 1 or 2MeV (million electronvolts). They are said to be fast neutrons. They must be slowed down in most reactors and become slow neutrons, to be able to cause a second fission and sustain the chain reaction.
Neutrons slow down when colliding with nuclei that ionize the surrounding matter while recoiling. The slowdown is fast in a moderator environment rich in hydrogen where the neutron can happen to lose all its energy in a single collision. The result of this series of collisions is an erratic path that can be long in air.
Once captured, the neutron usually does not emerge from the nucleus. The nucleus reacts by emitting a radiative gamma. The capture often leaves the nucleus in an unstable therefore radioactive state.
The probability – called cross section – of this capture varies greatly with the nucleus and the neutron energy. At characteristic energies (which corresponds to energy levels of the nucleus) the neutron resonates as it passes nearby and finds itself absorbed. It is as if the nucleus became suddenly very big. The phenomenon reminds of what happens in a musical instrument that resonates at certain frequencies. The neutron energy plays here the role of frequency.
In a conventional nuclear reactor, one must not lose inadvertently neutrons. During the slowdown, the resonant energies should be avoided together with nuclei which have too much appetite for neutrons. The choice of materials becomes critical.
The neutron flux is abundant and dangerous near the core of nuclear reactors. The phenomenon of resonant capture is then welcomed for radioprotection. Nuclei, such as, boron-10 or cadmium fond of slow neutrons are intentionally included in control rods and shielding materials. Similarly, one controls the reactor reactivity when the nuclear fuel is new by adding boron in the moderator water. This “boric” water tempers the fission reactions. The amount of boron-10 decreases during operation, together with the reactor, whose fuel becomes depleted, has less and less need to be bridled.
Neutron radiation effects remain after a capture. An isotope that contains an extra neutron is, moreover, generally unstable. Irradiated support structures in reactors become radioactive. Neutron captures in the fuel generate also radioactive nuclei heavier than uranium, such as plutonium isotopes and minor actinides. Radioisotopes useful for applications are also extracted from reactors.
Articles on the subject « Neutronic Radiations »
Neutron Capture
Capture competes with fission and generates radioactivity The neutron is a special elementary par[...]
Neutron Balance (fission)
What happens to fission neutrons? Within a reactor the objective is to maintain the chain reactio[...]
Slow and fast neutrons
Fission depends on the energy of the neutrons Nuclear fission can occur when a nucleus is rendere[...]
Slow neutrons
Slow neutrons favour fission In nuclear reactors slow neutrons are neutrons slowed down after a s[...]
Fast neutrons
Fast neutrons for surgeneration and breeders Before they are slowed down by a large number of nuc[...]
Neutron Moderators
Slowing fast neutrons without capturing them The presence of a moderator; is essential for operat[...]