The remarkable story of the neutrino
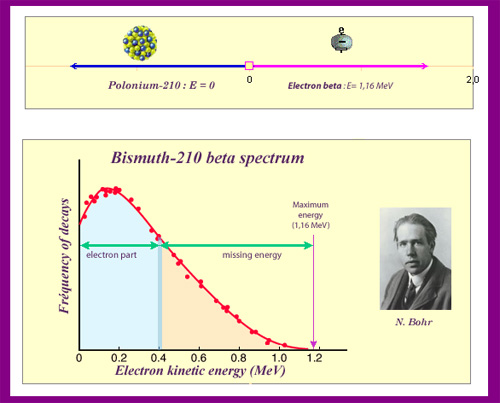
The enigma of the missing energy of beta électrons
Around 1930, beta decay was considered to produce two particles: the recoiling nucleus and an electron. Like in alpha decay, it was supposed to be a ‘monokinetic’ reaction, with the electron carrying almost all the available kinetic energy. Beta decay, however, produced a wide spectrum of possible and smaller energies for the electron, as the above figure shows. Where did the missing energy go? When confronted with this dilemma, Niels Bohr went so far as to ask himself whether or not the law of conservation of energy was still valid.
© IN2P3
The study of radioactive disintegrations had established that alpha rays were ‘monokinetic’ – in that they always took away the same percentage (100%) of the energy available in the decay. Beta rays, by comparison, could have any of a range of energy values. Whereas the alpha energy distribution is reduced to a single value, the energy distribution (or spectrum) of beta rays can take any value between zero and the total energy available.
In 1930, this evidence was problematic for physicists working in the field. What happened to the law of conservation of energy for beta decay? The seemingly missing energy even led Niels Bohr to propose doing away with that most fundamental conservation law. A mortal sin for a physicist.
In order to save the law of conservation of energy, the Swiss physicist Wolfgang Pauli postulated the existence of an electrically-neutral, low mass (at most 1/100 the mass of the proton) particle that would be emitted along with the beta particle. This hypothetical third body escaped detection but could then take away whatever energy was not given to the beta particle; solving that most vexing of issues. Pauli first proposed this hypothesis in a humorous letter to his colleagues Lise Meitner and Hans Geiger.
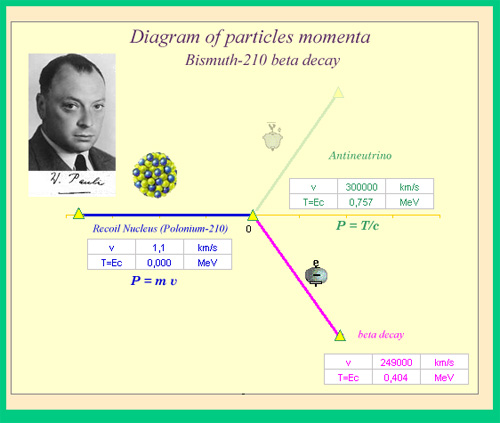
Resolving the issue by adding a new particle
It would be Wolfgang Pauli who resolved the issue of the missing energy by proposing the existence of a third particle capable escaping detection: the neutrino. Taking the example of bismuth 210 decay, the figure above shows the situation where all three bodies have equal momenta. The recoiling nucleus, some 400,000 times heavier than the electron, takes away a negligible amount of energy. The remaining energy is divided between the electron and the neutrino, shown here as a ghost particle. It took almost a quarter of a century for the first experimental evidence of a neutrino as a particle.
© IN2P3
Enrico Fermi, the great Italian physicist, was immediately convinced. Building on the discussions held at the Solvay Conference on October 1933 (devoted to James Chadwick’s discovery of the neutron), he proposed the theory of beta decay based on a hypothesis that an electron-neutrino pair is spontaneously produced by a nucleus in the same way that photons can spontaneously be emitted by excited atoms.
Francis Perrin concluded that for such a particle to exist it must either have zero mass or be lightweight even by comparison with the electron.
Fermi’s theory was the precursor of today theory of ‘weak interaction’. The Fermi’s theory of a point-like interaction of four particles (for instance, an initial neutron becoming a proton, an electron and an antineutrino), was accepted as an article of faith without proof during a quarter of century …
The neutrino remained a hypothetical particle until evidence for its existence was brought forward by Reines and Cowan in 1956, who were the first physicists to observed neutrinos.
In 1955, the American physicist Murray Gell Mann introduced the notion of a weak force active not only in radioactive processes, but in other processes whose effects involving several types of neutrinos could be seen in a host of subatomic phenomena.
Since its ‘invention’ by Pauli and the subsequent experimental evidence found in its favour, the neutrino and its antiparticle have played a vital role in the fundamentals of particle physics. Possessing no electrical charge, neutrinos are affected solely by the weak interaction, which allows for closer examination of that force without the ‘background noise’ provided by the other nuclear or atomic forces.
Besides the crucial role it plays in the physics of the infinitely small, the neutrino plays an important role in astrophysics. It could be for instance a key to understand the ‘hidden mass of the Universe’.
TO KNOW MORE ABOUT THE NEUTRINO DISCOVERY :
– 1956 : the experimental discovery
Other articles on the subject « Discoveries »
Marie Curie
Marie Sklodowska-Curie had an exceptional destiny. Born in Poland, she came to graduate school in[...]
Three radiations
Understanding the nature of alpha, beta and gamma rays In the years following the discovery of ra[...]
Discovery of the Nucleus
A new vision of the atom In 1911, Rutherford, Marsden and Geiger discovered the dense atomic nucl[...]
Ernest Rutherford
In October 1895, landed in England a 24 years-old young New Zealander. His name was Ernest Ruther[...]
Rutherford’s experiment
The experiment which proved the existence of a nucleus in the atom In 1908, Ernest Rutherford rec[...]
The neutron : Chadwick
A close competition between great physicists … James Chadwick, who discovered the neutron i[...]
Radium and Medicine
A short history: first steps in nuclear medicine … Everyone knows that the discovery of rad[...]
1934 : Artificial Radioactivity
The production at will of radioactive isotopes In the first days of 1934, Frederic and Irene Joli[...]
Fission discovery
A nuclear phenomenon that escaped the hands of physicists In the years spanning 1934 to 1938, Enr[...]
Enrico Fermi
A genial experimenter and theoretician Enrico Fermi (Rome, September 29, 1901 – Chicago, No[...]