Uranium 233: a fissile nucleus made from thorium
Thorium is more abundant than uranium in the Earth’s crust. Therefore, the possibilities of using thorium based fuels in reactors has been considered since the beginning of nuclear power. A major obstacle is that thorium, made of almost 100% thorium-232, is not fissile but only “fertile”. The capture of a neutron by a thorium-232 nucleus does not induce fission but transforms later on this thorium-232 into a uranium-233 nucleus. that is fissile. This transformation is similar to that of uranium-238 nuclei into fissile plutonium-239 within the fuels of current reactors.
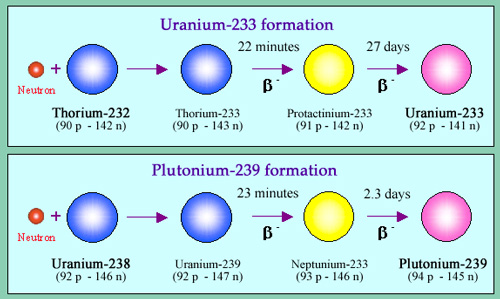
Uranium-233 and plutonium-239 formations
The uranium-233 formation from a fertile thorium-232 nucleus(top) is similar to the plutonium-239 formation from uranium-238 (below) in conventional reactors. A neutron capture leads to unstable thorium-233 formation that by two successive beta decays transforms itself into uranium-233. The main difference between the two mechanisms is that the protactinium-233 decay (into uranium-233), is 12 times (27-day period) slower than that of neptunium-239 into plutonium-239. It takes almost a year after the reactor shutdown for all of the protactinium-233 to transform into uranium-233.
© IN2P3
Uranium-233 has an extremely long half-life of 159,000 years, but too short for be still present on Earth. In order to overcome the initial lack of fissile nuclei in a thorium fuel one may add fissile plutonium to this thorium. Neutrons coming from plutonium, will be captured by thorium and transform this nuclei in uranium-233. Over time, this uranium-233 will gradually replacie the initial plutonium load.
This necessity of adding fissile elements to the thorium fuel to start such reactors has long been prohibitive. The renewed interest for the thorium cycle is due to the fact that large amounts of plutonium – coming from PWR and BWR reactors – have become available. Only a fraction of this plutonium is recycled with MOX fuels. Plutonium stocks can be used for the starting up of thorium reactors, where they would be burned.
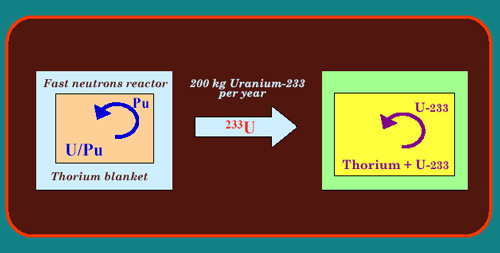
Starting thorium-uranium-233 reactors
Possible scenario for starting a molten salt thorium reactor. On the left, the core of a breeder reactor, loaded with enriched plutonium fuel and surrounded by a cover of fertile thorium, would generate about 200 kg per year of uranium-233. It would take several years of operation to reach the fissile charge of uranium-233, necessary to start a reactor (right) with molten salts of thorium. Then, this reactor would be able to regenerate the uranium-233 consumed.
© IN2P3
Once the initial obstacle overcome, Uranium-233 offers advantages from a nuclear standpoint, with respect to uranium-235 and plutonium-239, its fissile competitors. The losses of neutron captures not followed by fission are smaller. Neutron captures by uranium-233 leading to fissions produce a higher number of secondary neutrons : 2.28 neutrons per neutron captures, against 2.07 for uranium-235 and 2.11 for plutonium-239, in the case of slow neutrons. This excess would allow breeding not only with fast neutrons but also with slow neutrons, unlike plutonium breeder reactors that require fast nutrons. The deployment of thorium-uranium breeders would also require a smaller initial load of fissile nuclei.
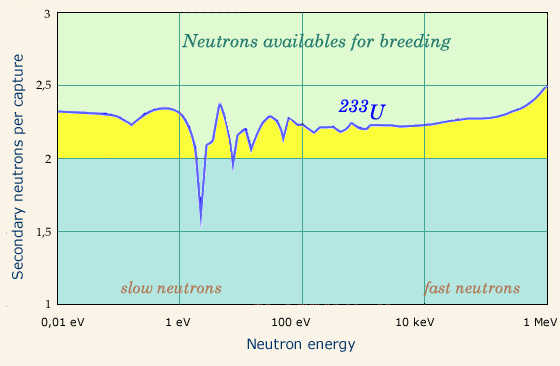
Uranium-233 breeding with slow and fast neutrons
The fission of uranium-233 produces more than 2 secondary neutrons per capture. If one of these neutrons generates a new fission, and a second is absorbed by a thorium-232 nucleus which transforms later on in uranium-233, the fissile fuel is regenerated. The advantage of uranium-233 is to allow breeding with slow and fast neutrons as well.
© IN2P3 (Source A.Nuttin/LPSC Grenoble)
Uranium-233 is considered “cleaner” than fuels based on uranium-235 and plutonium-239 (*). In conventional reactors, neutron captures lead to the formation of transuranic nuclei, minor actinides, which are the major contributors to the long term radiotoxicity of nuclear radioactive waste. In the case of uranium-233, neutron captures non followed by fissions lead instead to the formation of “transthorians” nuclei such as uranium-234, 235 and 236 which much longer half lives (247 000, 700 and 24 million years respectively). These tranthorians are therefore much less radioactive and toxic than minor actinides and plutonium isotopes.
Thorium reactors designs are often associated with a liquid core containing molten salts into which fissile materials would be introduced. This would eliminate fuel handling problems and the need for reloading. Continuous reprocessing would remove fission products from liquid materials making them less radioactive. One could add to the molten salts minor actinides and plutonium which over time would be destroyed. The reactor would then act as a waste burner.
If Molten Salt Reactors (MSR) are deployed in the future, they will likely be fast spectrum reactors. Fast reactors have the advantage of being able to operate with any fissile material which facilitates their start-up. The thorium + uranium-233 mode would then be reached not initially but in the long term.
Thorium fuel cycle reactors are one of the options considered for the Generation IV reactors that may replace in a far future today pressurized and boilling water reactors. Thorium is more abundant than uranium. A thorium cycle may ensure in principle the energy future of mankind for thousands of years. Furthermore, the long-term legacy of radioactive waste would be diminished by the absence of plutonium and actinides in thorium-uranium spent fuels. Thorium reactors may even be used to burn a part of the existings stocks of plutonium.
Other articles on the subject « Nuclear Fuel »
Nuclear Fuel Cycle
The front-end and the back-end of the cycle… The nuclear fuel cycle includes all nuclear op[...]
Cycle Front End
From the extraction of uranium to the fuel fabrication The front-end of the nuclear cycle is the [...]
Isotopic Separation
The access key to the fuel of modern nuclear power plants … and to atomic weapons The uranium fue[...]
Ultracentrifugation
A separation process economical in energy, but proliferating The principle of centrifuges has lon[...]
Gaseous Diffusion
The first enrichment process, a large consumer of electricity The gaseous diffusion process was t[...]
PWR fuel assemblies
Fuel assemblies in the core of PWR Reactors The core of a pressurized water reactor (PWR) is cont[...]
Reactor fuel layout
Fuel assemblies in the core of nuclear reactors The nuclear fuel assemblies, that vary from one r[...]
Plutonium Use
A fissile element produced in nuclear reactors In February 1941 the American physicist Glenn Seab[...]
Pu : Fuel, bomb or Waste ?
Plutonium dark and bright sides … Sensitive material, like the roman god Janus, plutonium h[...]
MOX fuels
Introducing plutonium in nuclear fuels For energy production, plutonum is gold ! One gram of plut[...]