Irradiation rate and energy supplied by fuel
The energy produced by a nuclear power plant is proportional to the irradiation to which the fuel in the reactor core is subjected. This relationship is expressed by the “burn-up rate“. One tonne of uranium is able to cover the electricity requirements of a city with a population of 50,000 for three years. In a PWR reactor, uranium releases 33 gigawatt-days (792 million kilowatt-hours) of energy per tonne in standard operating conditions. Electricity companies express power output from their plants in terms of gigawatt-days rather than kilowatt-hours. One gigawatt-day equals 24 million kilowatt-hours, i.e. 1 million kilowatts during 24 hours.
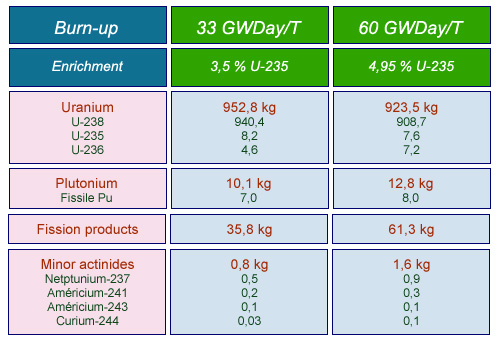
Influence of burn-up on fuel composition
The table gives a comparison of the composition of spent fuel after removal from the reactor of two fuels, respectively enriched to 3.5 and 4.95% uranium-235 after they have delivered 33 and 60 GWd energy per initial tonne of UO2 fuel.
© IN2P3 (source CEA)
As the fuel supplies energy, its fissile uranium-235 is depleted. Eventually, it must be removed from the reactor. Power generators and engineers seek to delay this requirement in order to increase the power generated and hence the burn-up. This goal can be achieved by more highly enriching the fuel with uranium-235. However, the irradiation resistance of fuel cladding that will be irradiated for extended periods must be enhanced.
The composition of the fuel upon removal from the reactor depends on the burn-up, as illustrated in the example above, which shows the composition of two spent fuels, one enriched to 3.5% and used to generate 36 gigawatt-days/tonne, the other enriched to 4.95% and used to generate 60 gigawatt-days per tonne.
The second fuel generated 88% more energy. This near-doubling of the burn-up results in a proportionate increase in fission products and actinides. The amount of plutonium increased by only 27%, however. This is because an extended stay in the reactor allows time for the plutonium formed in the fuel to be subsequently consumed by fission. The plutonium thus consumed contributes to the power output.
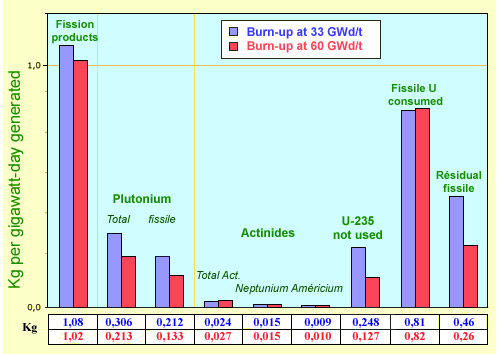
Effects of a higher burn-up
The diagram shows the effects of reactor residence time for a constant quantity of energy produced. It compares the composition upon removal from the reactor of a fuel used to generate 60 GigaWatt-day/ton and a standard fuel delivering 33 GWday/ton. These contents are restated for an equivalent energy output of 1 GWd. At equivalent energy production, the quantities of fissile uranium 235 burned and fission products generated are essentially the same. However, there is 30% less plutonium and the unburned residual fissile material (U-235 and Pu-239) content is 44% lower.
© Source: CEA
Prolonged irradiation periods (4 years or more) offer benefits from a plant operation perspective, as they enable reactors to consume less uranium-235 and produce less plutonium for a given energy output. Companies such as EDF are increasingly adopting these longer fuel cycles as a means of reducing their plutonium inventories.
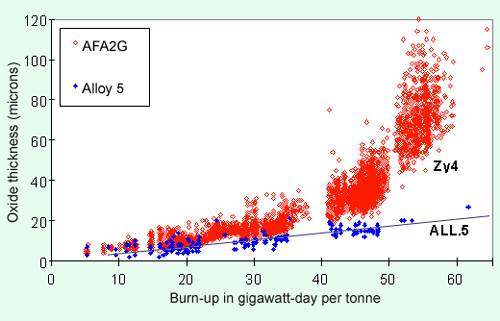
Technical advances enabling burn-up increase
Technical advances are enabling the degree of irradiation of nuclear fuel to be increased. The zirconium alloys used in fuel cladding (measurements shown in red) oxidise in the presence of water. This phenomenon is greatly exacerbated at burn-up rates exceeding 50 GWd/t, limiting the fuel’s residence time and the reactor’s energy performance. Improved cladding alloys (measurements shown in blue) can be used to considerably reduce the thickness of the oxide (i.e. corrosion) layer and achieve higher burn-up values.
© AREVA/Framatome
Certain limitations prevent burn-up levels from being pushed very far in water reactors. Above 50 gigawatt-day/tonne, alternative alloys to zircaloy-4 must be used, to prevent corrosion of cladding in water, resulting in the release of radioactive gaseous fission products. At these levels, structural strain in fuel assemblies becomes apparent, creating a risk of control rods becoming jammed.
Other articles on the subject « Spent nuclear fuel »
Reactor unloading
Fuel unloading and initial pool storage of spent fuel The fuel load in a conventional pressurised[...]
Spent fuel composition
Key figures on spent fuel composition The composition of irradiated fuel removed from a reactor c[...]
Waste radioactivity decrease
A natural but slow decay process As natural radioactivity progressively decays, the radioactivity[...]
Spent fuel decay heat
Significant, slow-to-decline heat release The heat released by radioactive disintegration is a ke[...]
Spent fuel radiotoxicity
A risk indicator more pessimistic than radioactive activity Radioactivity is an imperfect descrip[...]