Area 1: Transmuting or transforming radioactive atoms
Transmutation is the second part of research area 1 under the Bataille and 2006 Acts, following on naturally from partitioning. Researchers will seek to transmute the minor actinides such as neptunium, americium and curium, which contribute most to the radioactive toxicity of waste over the long term, and possibly long-lived fission products such as technetium.
Unlike the partitioning, disposal, conditioning and storage research areas, which use proven industrial techniques, transmutation is a much more difficult and delicate technique because it involves altering the nucleus of an atom. It is a nuclear technique. Transmutation is done atom by atom, and there are a lot of atoms to transmute.
Transmutation consists of changing the nature of the nuclei of atoms by means of nuclear reactions caused by neutrons: fission reactions or simple neutron capture. This is how some of the uranium in the core of a reactor is converted into fission products or, by successive neutron captures, into plutonium or the minor actinides.
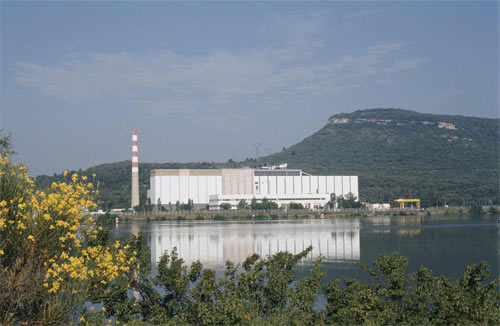
The PHENIX reactor: a transmutation facility
Located on the banks of the Rhône on the nuclear site at Marcoule in Gard, PHENIX is a prototype power plant with a sodium-cooled fast reactor, a rare reactor type. It is France’s main facility for carrying out studies of transmutation. PHENIX went into operation in 1973 and was upgraded in 1998 for the purpose of carrying out research, following the decommissioning of SUPERPHENIX, until October 2009 when it was also decommissioned.
© A.Gonin/CEA
A difficult technique with low efficiency levels
The principle consists of destroying long-lived elements that have previously been partitioned, by turning them into stable, short-lived or medium-lived radioelements. Though it may seem attractive on paper, in practice it is very difficult: only 5% of the uranium in a reactor is transmuted after 3 years!
To improve this efficiency, the neutrons have to have enough energy that their probability of interacting, known as their cross section, is as high as possible, and there must be a high neutron flow. For transmutation, research will therefore be done into special reactors using fast neutrons with high flows, because fast neutrons are better able to cause the fission of the minor actinides.
The transmutable elements are transformed by neutron capture, which may or may not be followed by fission. Fission, which breaks the actinides into stable or short-lived fragments, supplies neutrons. Conversely, transforming fission products consumes neutrons.
For transmutation to be effective, high neutron flows are necessary. The radioelements to be transmuted would be inserted into the reactor fuel in the form of a target or diluted in the fuel homogeneously.
There are two transmutation methods. The first uses fast neutron reactors (conventional reactors are not very effective) and the second uses hybrid reactors (Accelerator-Driven Systems or ADSs) such as the MYHRRA project. Because they use neutrons from an outside source, in principle ADSs can burn any waste including long-lived fission products.
French law sees transmutation as the ultimate objective of waste management: the stuff of dreams but difficult to achieve. No suitable reactors yet exist. France’s main facility was PHENIX, a fairly old fast reactor that was upgraded in 1998 but decommissioned in 2009. Since PHENIX was decommissioned, French researchers have not had access to any experimental facilities in mainland France. They are having to use foreign facilities until the ASTRID fast reactor for demonstration is available from 2020.
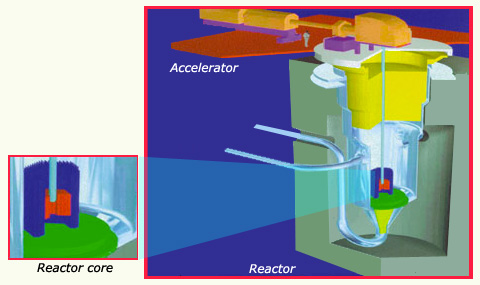
Transmutation: the hybrid reactor or ADS option
In the framework of the future Generation IV fast reactors program, France is also taking part in a study of hybrid reactors that can transmute actinides and long-lived fission products and therefore reduce their radiotoxicity. These reactors would combine a particle accelerator, which would provide a constant source of neutrons, with a subcritical reactor, which would multiply these neutrons to transmute the more troublesome radionuclides.
© CEA
The transmutation of certain minor actinides, such as americium and neptunium, has been demonstrated scientifically. However, the transmutation of curium, which is highly radioactive and difficult to handle, continues to pose major problems.
According to the French National Assessment Board (CNE), the feasibility of manufacturing nuclear materials suitable for the large-scale transmutation of minor actinides has not been demonstrated either. A report by CEA identifies the difficulties that need to be overcome first and a number of tests are in progress or pending. The feasibility of some Generation IV reactor types and the ADSs depends on them. The difficulties associated with incorporating curium into nuclear materials remain unresolved.
The first demonstration facilities of the Generation IV reactors and ADSs designed specifically for transmutation are expected in the 2020-2030 period. A very active international cooperation involving Russia, Japan, the US and many European countries has been set up. Taking account of the time it takes to develop these facilities and of the checks to be carried out, the transmutation of minor actinides on an industrial scale can be envisaged in 2040 at the earliest.
NEXT : Transmutation facilities
NEXT : Transmutation prospects
Other articles on the subject « Waste Outlooks »
Three research areas
Three complementary research areas The three research areas under the Bataille Act, later supplem[...]
Area 1: Partitioning
Sorting the radioelements in radioactive waste The separation, or partitioning, of the radioactiv[...]
Partitioning: results
Development of processes and extractant molecules The 2006 assessment of research into partitioni[...]
Advanced partitioning
Partitioning of nuclear waste: hot chemistry Advanced partitioning goes beyond just the partition[...]
Transmutation facilities
Fast reactors and Accelerator Driven Systems (ADS) The neutron, which easily penetrates nuclei, i[...]
Transmutation prospects
A far away objective for Generation IV and 2040… At the time of the first tests on the tran[...]
Area 2: Disposal
Research into deep geological disposal Can the nuclear industry’s final waste, which we cur[...]
Area 3: Long Surface Storage
Research into long-term storage Research into long-term storage is the second research topic in a[...]
Long surface storage concepts
Long-term surface and subsurface storage Research into very long-term storage is a matter for the[...]
Area 3: Conditioning
Area 3: Research into conditioning The conditioning of high-level and intermediate-level waste is[...]